Получена: 22 сентября 2023/ Принята: 16 ноября/ Опубликована online: 28 декабря 2023
УДК: 577.24
DOI: /10.26212/2227-1937.2023.55.94.017
А.С. Жакупова1, ORCID: http://orcid:0000-0003-4950-160X
А.А. Турмухамбетова2, ORCID: http://orcid:0000-0002-4668-3295
Т.М. Салиев3, ORCID: http://orcid:0000-0001-5696-6363
П.Б. Сингх1, ORCID: http://orcid:0000-0002-9571-0974
1 Школа Медицины Назарбаев Университет, г. Астана
2 НАО «Медицинский университет Караганды», г. Караганды
3 Научно-исследовательский институт фундаментальной и прикладной медицины имени Б. Атчабарова (НИИФПМ), г.Алматы
ПОНИМАНИЕ КОНЦЕПЦИИ ПЕРЕПРОГРАММИРОВАНИЯ ВОЗРАСТА
Резюме: Перепрограммирование возраста представляет собой новый метод создания пациент-специфичных тканей
для трансплантации. Данный метод позволяет обойти цикл повторной дифференцировки, характерный для
индуцированных плюрипотентных стволовых клеток (iPS) и ядерно-трансферных эмбриональных стволовых
клеток (NT-ES). Данные технологии вызывают большой интерес в регенеративной медицине. Несмотря на
очевидный потенциал клеточной терапии iPS и NT-ES, существует несколько проблем, которые необходимо
преодолеть, прежде чем данные методы лечения станут безопасными и рутинными. Альтернативой
вышеперечисленным методам является перепрограммирование возраста, которое направлено на омоложение
специализированных функций старой клетки без прохождения де-дифференцировки. Также в отличие от клеточной
терапии iPS и NT-ES, перепрограммирование возраста не требует прохождения перепрограммирования развития
через эмбриональную стадию. Работы по перепрограммированию возраста были в основном сосредоточены на
одном аспекте – эпигеноме. Эпигенетическое омоложение было достигнуто in vitro в отсутствии дедифференцировки с использованием факторов перепрограммирования iPS клеток. Исследования динамики
перепрограммирования эпигенетического возраста (eAge) показали, что отличие eAge от перепрограммирования
развития может быть в значительной степени объяснено их различной кинетикой. Перепрограммирование
возраста также было достигнуто in vivo и было показано, что оно увеличивает продолжительность жизни у мышей с
преждевременным старением. В данной статье приводятся доказательства того, что перепрограммирование
развития можно регулировать in vitro и in vivo, а также направления будущих исследований для углубления нашего
понимания данного явления, которое даст значительные преимущества в регенеративной терапии.
Ключевые слова: перепрограммирование возраста, эпигенетическое омоложение, перенос ядер соматических
клеток (SCNT), iPS клетки, факторы перепрограммирования, эпигенетические часы, eAge.
СПИСОК ЛИТЕРАТУРЫ
1 Briggs, R., and King, T.J. (1952). Transplantation of
living nuclei from blastula cells into enucleated frogs’
eggs. Proc. Natl. Acad. Sci. U.S.A. 38, 455–463.
10.1073/pnas.38.5.455.
2 Gurdon, J.B. (1962). Adult frogs derived from the nuclei
of single somatic cells. Developmental Biology 4, 256–273.
10.1016/0012-1606(62)90043-X.
3 Wilmut, I., Schnieke, A.E., McWhir, J., Kind, A.J., and
Campbell, K.H.S. (1997). Viable offspring derived from
fetal and adult mammalian cells. Nature 385, 810–813.
10.1038/385810a0.
4 Mizutani, E., Ono, T., Li, C., Maki-Suetsugu, R., and
Wakayama, T. (2008). Propagation of senescent mice
using nuclear transfer embryonic stem cell lines. genesis
46, 478–483. 10.1002/dvg.20420.
5 Wakayama, S., Mizutani, E., and Wakayama, T. (2010).
Production of Cloned Mice from Somatic Cells, ES Cells,
and Frozen Bodies. In Methods in Enzymology (Elsevier),
pp. 151–169. 10.1016/S0076-6879(10)76009-2.
6 Dimos, J.T., Rodolfa, K.T., Niakan, K.K., Weisenthal, L.M.,
Mitsumoto, H., Chung, W., Croft, G.F., Saphier, G., Leibel, R.,
Goland, R., et al. (2008). Induced Pluripotent Stem Cells
Generated from Patients with ALS Can Be Differentiated
into Motor Neurons. Science 321, 1218–1221.
10.1126/science.1158799.
7 Takahashi, K., and Yamanaka, S. (2006). Induction of
Pluripotent Stem Cells from Mouse Embryonic and Adult
Fibroblast Cultures by Defined Factors. Cell 126, 663–676.
10.1016/j.cell.2006.07.024.
8 Lapasset, L., Milhavet, O., Prieur, A., Besnard, E., Babled,
A., Aït-Hamou, N., Leschik, J., Pellestor, F., Ramirez, J.-M.,
De Vos, J., et al. (2011). Rejuvenating senescent and
centenarian human cells by reprogramming through the
pluripotent state. Genes Dev. 25, 2248–2253.
10.1101/gad.173922.111.
9 López-Otín, C., Blasco, M.A., Partridge, L., Serrano, M.,
and Kroemer, G. (2013). The Hallmarks of Aging. Cell 153,
1194–1217. 10.1016/j.cell.2013.05.039.
10 López-Otín, C., Blasco, M.A., Partridge, L., Serrano, M.,
and Kroemer, G. (2023). Hallmarks of aging: An
expanding universe. Cell 186, 243–278.
10.1016/j.cell.2022.11.001.
11 Marion, R.M., Strati, K., Li, H., Tejera, A., Schoeftner, S.,
Ortega, S., Serrano, M., and Blasco, M.A. (2009). Telomeres
Acquire Embryonic Stem Cell Characteristics in Induced
Pluripotent Stem Cells. Cell Stem Cell 4, 141–154.
10.1016/j.stem.2008.12.010.
12 Schaetzlein, S., Lucas-Hahn, A., Lemme, E., Kues, W.A.,
Dorsch, M., Manns, M.P., Niemann, H., and Rudolph, K.L.
(2004). Telomere length is reset during early mammalian
130
embryogenesis. Proc. Natl. Acad. Sci. U.S.A. 101, 8034–
8038. 10.1073/pnas.0402400101.
13 Liu, L., Bailey, S.M., Okuka, M., Muñoz, P., Li, C., Zhou, L.,
Wu, C., Czerwiec, E., Sandler, L., Seyfang, A., et al. (2007).
Telomere lengthening early in development. Nat Cell Biol
9, 1436–1441. 10.1038/ncb1664.
14 Marión, R.M., Strati, K., Li, H., Murga, M., Blanco, R.,
Ortega, S., Fernandez-Capetillo, O., Serrano, M., and
Blasco, M.A. (2009). A p53-mediated DNA damage
response limits reprogramming to ensure iPS cell
genomic integrity. Nature 460, 1149–1153.
10.1038/nature08287.
15 Suhr, S.T., Chang, E.A., Tjong, J., Alcasid, N., Perkins,
G.A., Goissis, M.D., Ellisman, M.H., Perez, G.I., and Cibelli,
J.B. (2010). Mitochondrial Rejuvenation After Induced
Pluripotency. PLoS ONE 5, e14095.
10.1371/journal.pone.0014095.
16 Herberts, C.A., Kwa, M.S., and Hermsen, H.P. (2011).
Risk factors in the development of stem cell therapy. J
Transl Med 9, 29. 10.1186/1479-5876-9-29.
17 Abad, M., Mosteiro, L., Pantoja, C., Cañamero, M.,
Rayon, T., Ors, I., Graña, O., Megías, D., Domínguez, O.,
Martínez, D., et al. (2013). Reprogramming in vivo
produces teratomas and iPS cells with totipotency
features. Nature 502, 340–345. 10.1038/nature12586.
18 Ohnishi, K., Semi, K., Yamamoto, T., Shimizu, M.,
Tanaka, A., Mitsunaga, K., Okita, K., Osafune, K., Arioka, Y.,
Maeda, T., et al. (2014). Premature Termination of
Reprogramming In Vivo Leads to Cancer Development
through Altered Epigenetic Regulation. Cell 156, 663–677.
10.1016/j.cell.2014.01.005.
19 Manukyan, M., and Singh, P.B. (2012). Epigenetic
rejuvenation. Genes to Cells 17, 337–343. 10.1111/j.1365-
2443.2012.01595.x.
20 Singh, P.B., and Zacouto, F. (2010). Nuclear
reprogramming and epigenetic rejuvenation. J Biosci 35,
315–319. 10.1007/s12038-010-0034-2.
21 Singh, P.B., and Zhakupova, A. (2022). Age
reprogramming: cell rejuvenation by partial
reprogramming. Development 149, dev200755.
10.1242/dev.200755.
22 Manukyan, M., and Singh, P.B. (2014). Epigenome
rejuvenation: HP1β mobility as a measure of pluripotent
and senescent chromatin ground states. Sci Rep 4, 4789.
10.1038/srep04789.
23 Horvath, S. (2013). DNA methylation age of human
tissues and cell types. Genome Biology 14, R115.
10.1186/gb-2013-14-10-r115.
24 Armstrong, N.J., Mather, K.A., Thalamuthu, A., Wright,
M.J., Trollor, J.N., Ames, D., Brodaty, H., Schofield, P.R.,
Sachdev, P.S., and Kwok, J.B. (2017). Aging, exceptional
longevity and comparisons of the Hannum and Horvath
epigenetic clocks. Epigenomics 9, 689–700. 10.2217/epi2016-0179.
25 Vanyushin, B.F., Nemirovsky, L.E., Klimenko, V.V.,
Vasiliev, V.K., and Belozersky, A.N. (1973). The 5-
methylcytosine in DNA of rats. Tissue and age specificity
and the changes induced by hydrocortisone and other
agents. Gerontologia 19, 138–152.
26 Jung, M., and Pfeifer, G.P. (2015). Aging and DNA
methylation. BMC Biol 13, 7. 10.1186/s12915-015-0118-
4.
27 Bell, C.G., Lowe, R., Adams, P.D., Baccarelli, A.A., Beck,
S., Bell, J.T., Christensen, B.C., Gladyshev, V.N., Heijmans,
B.T., Horvath, S., et al. (2019). DNA methylation aging
clocks: challenges and recommendations. Genome Biol 20,
249. 10.1186/s13059-019-1824-y.
28 Mendelsohn, A.R., and Larrick, J.W. (2019). Epigenetic
Age Reversal by Cell-Extrinsic and Cell-Intrinsic Means.
Rejuvenation Research 22, 439–446.
10.1089/rej.2019.2271.
29 Bogdanović, O., Smits, A.H., de la Calle Mustienes, E.,
Tena, J.J., Ford, E., Williams, R., Senanayake, U., Schultz,
M.D., Hontelez, S., van Kruijsbergen, I., et al. (2016). Active
DNA demethylation at enhancers during the vertebrate
phylotypic period. Nat Genet 48, 417–426.
10.1038/ng.3522.
30 Field, A.E., Robertson, N.A., Wang, T., Havas, A., Ideker,
T., and Adams, P.D. (2018). DNA Methylation Clocks in
Aging: Categories, Causes, and Consequences. Molecular
Cell 71, 882–895. 10.1016/j.molcel.2018.08.008.
31 Unnikrishnan, A., Freeman, W.M., Jackson, J., Wren, J.D.,
Porter, H., and Richardson, A. (2019). The role of DNA
methylation in epigenetics of aging. Pharmacology &
Therapeutics 195, 172–185.
10.1016/j.pharmthera.2018.11.001.
32 Hannum, G., Guinney, J., Zhao, L., Zhang, L., Hughes, G.,
Sadda, S., Klotzle, B., Bibikova, M., Fan, J.-B., Gao, Y., et al.
(2013). Genome-wide Methylation Profiles Reveal
Quantitative Views of Human Aging Rates. Molecular Cell
49, 359–367. 10.1016/j.molcel.2012.10.016.
33 Lu, A.T., Fei, Z., Haghani, A., Robeck, T.R., Zoller, J.A., Li,
C.Z., Lowe, R., Yan, Q., Zhang, J., Vu, H., et al. (2023).
Universal DNA methylation age across mammalian
tissues. Nat Aging. 10.1038/s43587-023-00462-6.
34 Horvath, S., and Raj, K. (2018). DNA methylation-based
biomarkers and the epigenetic clock theory of ageing. Nat
Rev Genet 19, 371–384. 10.1038/s41576-018-0004-3.
35 Gibbs, W.W. (2014). Biomarkers and ageing: The clockwatcher. Nature 508, 168–170. 10.1038/508168a.
36 Gladyshev, V.N. (2021). The Ground Zero of
Organismal Life and Aging. Trends in Molecular Medicine
27, 11–19. 10.1016/j.molmed.2020.08.012.
37 Olova, N., Simpson, D.J., Marioni, R.E., and Chandra, T.
(2019). Partial reprogramming induces a steady decline
in epigenetic age before loss of somatic identity. Aging
Cell 18, e12877. 10.1111/acel.12877.
38 Ohnuki, M., Tanabe, K., Sutou, K., Teramoto, I.,
Sawamura, Y., Narita, M., Nakamura, M., Tokunaga, Y.,
Nakamura, M., Watanabe, A., et al. (2014). Dynamic
regulation of human endogenous retroviruses mediates
factor-induced reprogramming and differentiation
potential. Proc. Natl. Acad. Sci. U.S.A. 111, 12426–12431.
10.1073/pnas.1413299111.
39 Ocampo, A., Reddy, P., Martinez-Redondo, P., PlateroLuengo, A., Hatanaka, F., Hishida, T., Li, M., Lam, D., Kurita,
M., Beyret, E., et al. (2016). In Vivo Amelioration of AgeAssociated Hallmarks by Partial Reprogramming. Cell
167, 1719-1733.e12. 10.1016/j.cell.2016.11.052.
40 Scaffidi, P., and Misteli, T. (2005). Reversal of the
cellular phenotype in the premature aging disease
Hutchinson-Gilford progeria syndrome. Nat Med 11, 440–
445. 10.1038/nm1204.
41 Simpson, D.J., Olova, N.N., and Chandra, T. (2021).
Cellular reprogramming and epigenetic rejuvenation. Clin
Epigenet 13, 170. 10.1186/s13148-021-01158-7.
42 Parras, A., Vílchez-Acosta, A., Desdín-Micó, G., Mrabti,
C., Rechsteiner, C., Battiston, F., Branchina, C., Pérez, K.,
Sempoux, C., and Ocampo, A. (2022). In vivo
reprogramming leads to premature death due to hepatic
and intestinal failure. bioRxiv, 2022.05.27.493700.
10.1101/2022.05.27.493700.
43 Hong, H., Takahashi, K., Ichisaka, T., Aoi, T., Kanagawa,
O., Nakagawa, M., Okita, K., and Yamanaka, S. (2009).
Suppression of induced pluripotent stem cell generation
131
by the p53–p21 pathway. Nature 460, 1132–1135.
10.1038/nature08235.
44 Singh, P.B., and Newman, A.G. (2018). Age
reprogramming and epigenetic rejuvenation. Epigenetics
& Chromatin 11, 73. 10.1186/s13072-018-0244-7.
45 Brown, J.P., Wei, W., and Sedivy, J.M. (1997). Bypass of
Senescence After Disruption of p21 CIP1/WAF1 Gene in
Normal Diploid Human Fibroblasts. Science 277, 831–
834. 10.1126/science.277.5327.831.
46 Gill, D., Parry, A., Santos, F., Okkenhaug, H., Todd, C.D.,
Hernando-Herraez, I., Stubbs, T.M., Milagre, I., and Reik,
W. (2022). Multi-omic rejuvenation of human cells by
maturation phase transient reprogramming. eLife 11,
e71624. 10.7554/eLife.71624.
47 Efe, J.A., Hilcove, S., Kim, J., Zhou, H., Ouyang, K., Wang,
G., Chen, J., and Ding, S. (2011). Conversion of mouse
fibroblasts into cardiomyocytes using a direct
reprogramming strategy. Nat Cell Biol 13, 215–222.
10.1038/ncb2164.
48 Nagy, A., and Nagy, K. (2010). The mysteries of induced
pluripotency: where will they lead? Nat Methods 7, 22–24.
10.1038/nmeth.f.292.
49 Guo, L., Karoubi, G., Duchesneau, P., Shutova, M.V.,
Sung, H.-K., Tonge, P., Bear, C., Rogers, I., Nagy, A., and
Waddell, T.K. (2017). Generation of Induced Progenitorlike Cells from Mature Epithelial Cells Using Interrupted
Reprogramming. Stem Cell Reports 9, 1780–1795.
10.1016/j.stemcr.2017.10.022.
50 Gurdon, J.B., and Melton, D.A. (2008). Nuclear
Reprogramming in Cells. Science 322, 1811–1815.
10.1126/science.1160810.
51 Yamanaka, S., and Blau, H.M. (2010). Nuclear
reprogramming to a pluripotent state by three
approaches. Nature 465, 704–712. 10.1038/nature09229.
52 Sarkar, T.J., Quarta, M., Mukherjee, S., Colville, A., Paine,
P., Doan, L., Tran, C.M., Chu, C.R., Horvath, S., Qi, L.S., et al.
(2020). Transient non-integrative expression of nuclear
reprogramming factors promotes multifaceted
amelioration of aging in human cells. Nat Commun 11,
1545. 10.1038/s41467-020-15174-3.
53 Lu, Y., Brommer, B., Tian, X., Krishnan, A., Meer, M.,
Wang, C., Vera, D.L., Zeng, Q., Yu, D., Bonkowski, M.S., et al.
(2020). Reprogramming to recover youthful epigenetic
information and restore vision. Nature 588, 124–129.
10.1038/s41586-020-2975-4.
54 Rodríguez-Matellán, A., Alcazar, N., Hernández, F.,
Serrano, M., and Ávila, J. (2020). In Vivo Reprogramming
Ameliorates Aging Features in Dentate Gyrus Cells and
Improves Memory in Mice. Stem Cell Reports 15, 1056–
1066. 10.1016/j.stemcr.2020.09.010.
55 Shahini, A., Rajabian, N., Choudhury, D., Shahini, S.,
Vydiam, K., Nguyen, T., Kulczyk, J., Santarelli, T., Ikhapoh,
I., Zhang, Y., et al. (2021). Ameliorating the hallmarks of
cellular senescence in skeletal muscle myogenic
progenitors in vitro and in vivo. Sci. Adv. 7, eabe5671.
10.1126/sciadv.abe5671.
56 Browder, K.C., Reddy, P., Yamamoto, M., Haghani, A.,
Guillen, I.G., Sahu, S., Wang, C., Luque, Y., Prieto, J., Shi, L.,
et al. (2022). In vivo partial reprogramming alters ageassociated molecular changes during physiological aging
in mice. Nat Aging 2, 243–253. 10.1038/s43587-022-
00183-2.
57 Chondronasiou, D., Gill, D., Mosteiro, L., Urdinguio, R.G.,
Berenguer‑Llergo, A., Aguilera, M., Durand, S.,
Aprahamian, F., Nirmalathasan, N., Abad, M., et al. (2022).
Multi‑omic rejuvenation of naturally aged tissues by a
single cycle of transient reprogramming. Aging Cell 21.
10.1111/acel.13578.
58 Roux, A.E., Zhang, C., Paw, J., Zavala-Solorio, J.,
Malahias, E., Vijay, T., Kolumam, G., Kenyon, C., and
Kimmel, J.C. (2022). Diverse partial reprogramming
strategies restore youthful gene expression and
transiently suppress cell identity. Cell Systems 13, 574-
587.e11. 10.1016/j.cels.2022.05.002.
59 Cheng, F., Wang, C., Ji, Y., Yang, B., Shu, J., Shi, K., Wang,
L., Wang, S., Zhang, Y., Huang, X., et al. (2022). Partial
reprogramming strategy for intervertebral disc
rejuvenation by activating energy switch. Aging Cell 21.
10.1111/acel.13577.
60 Alle, Q., Le Borgne, E., Bensadoun, P., Lemey, C., Béchir,
N., Gabanou, M., Estermann, F., Bertrand‑Gaday, C.,
Pessemesse, L., Toupet, K., et al. (2022). A single short
reprogramming early in life initiates and propagates an
epigenetically related mechanism improving fitness and
promoting an increased healthy lifespan. Aging Cell 21,
e13714. 10.1111/acel.13714.
61 Yang, J.-H., Hayano, M., Griffin, P.T., Amorim, J.A.,
Bonkowski, M.S., Apostolides, J.K., Salfati, E.L., Blanchette,
M., Munding, E.M., Bhakta, M., et al. (2023). Loss of
epigenetic information as a cause of mammalian aging.
Cell 186, 305-326.e27. 10.1016/j.cell.2022.12.027.
62 Tang, Y., Liu, M.-L., Zang, T., and Zhang, C.-L. (2017).
Direct Reprogramming Rather than iPSC-Based
Reprogramming Maintains Aging Hallmarks in Human
Motor Neurons. Front. Mol. Neurosci. 10, 359.
10.3389/fnmol.2017.00359.
63 Maza, I., Caspi, I., Zviran, A., Chomsky, E., Rais, Y.,
Viukov, S., Geula, S., Buenrostro, J.D., Weinberger, L.,
Krupalnik, V., et al. (2015). Transient acquisition of
pluripotency during somatic cell transdifferentiation with
iPSC reprogramming factors. Nat Biotechnol 33, 769–774.
10.1038/nbt.3270.
64 Chen, Y., Lüttmann, F.F., Schoger, E., Schöler, H.R.,
Zelarayán, L.C., Kim, K.-P., Haigh, J.J., Kim, J., and Braun, T.
(2021). Reversible reprogramming of cardiomyocytes to a
fetal state drives heart regeneration in mice. Science 373,
1537–1540. 10.1126/science.abg5159.
65 Hishida, T., Yamamoto, M., Hishida-Nozaki, Y., Shao, C.,
Huang, L., Wang, C., Shojima, K., Xue, Y., Hang, Y.,
Shokhirev, M., et al. (2022). In vivo partial cellular
reprogramming enhances liver plasticity and
regeneration. Cell Reports 39, 110730.
10.1016/j.celrep.2022.110730.
66 Wang, C., Rabadan Ros, R., Martinez-Redondo, P., Ma,
Z., Shi, L., Xue, Y., Guillen-Guillen, I., Huang, L., Hishida, T.,
Liao, H.-K., et al. (2021). In vivo partial reprogramming of
myofibers promotes muscle regeneration by remodeling
the stem cell niche. Nat Commun 12, 3094.
10.1038/s41467-021-23353-z.
67 Göbel, C., Goetzke, R., Eggermann, T., and Wagner, W.
(2018). Interrupted reprogramming into induced
pluripotent stem cells does not rejuvenate human
mesenchymal stromal cells. Sci Rep 8, 11676.
10.1038/s41598-018-30069-6.
68 Hou, P., Li, Y., Zhang, X., Liu, C., Guan, J., Li, H., Zhao, T.,
Ye, J., Yang, W., Liu, K., et al. (2013). Pluripotent Stem Cells
Induced from Mouse Somatic Cells by Small-Molecule
Compounds. Science 341, 651–654.
10.1126/science.1239278.
69 Guan, J., Wang, G., Wang, J., Zhang, Z., Fu, Y., Cheng, L.,
Meng, G., Lyu, Y., Zhu, J., Li, Y., et al. (2022). Chemical
reprogramming of human somatic cells to pluripotent
stem cells. Nature 605, 325–331. 10.1038/s41586-022-
04593-5.
70 Yang, J.-H., Petty, C.A., Dixon-McDougall, T., Lopez, M.V.,
Tyshkovskiy, A., Maybury-Lewis, S., Tian, X., Ibrahim, N.,
132
Chen, Z., Griffin, P.T., et al. (2023). Chemically induced
reprogramming to reverse cellular aging. Aging 15, 5966–
5989. 10.18632/aging.204896.
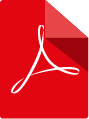